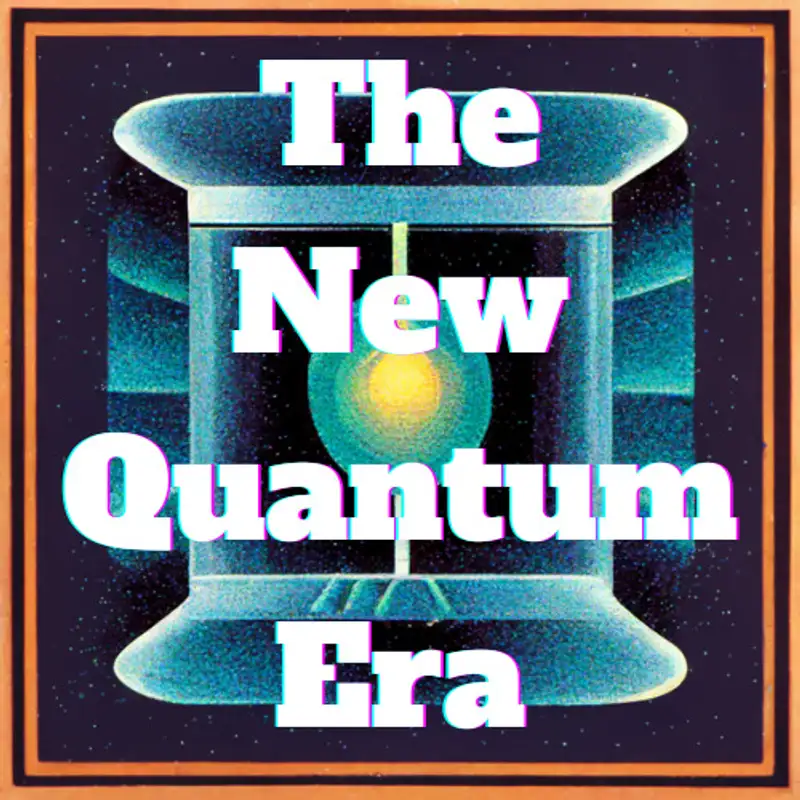
Fluxonium Qubits with Will Oliver
Welcome to the new quantum era. I'm your host, Sebastian Hassinger. I'm recording this at the American Physical Society's Global Summit, a conference that brings together 14,000 physicists from all over the world. As you might imagine, quantum information and quantum computing are very hot topics at this event in 2025. So I was really excited when the opportunity arose to spend the week here recording interviews with some of the best known researchers in the field and many new and upcoming talents.
Sebastian Hassinger:I want to thank the APS for providing me with space to record and to apologize, to the social media teams I had to kick out of the room. I don't have the luxury as writers do of sharing space when I'm recording, and I apologize for that. Today's episode is with Will Oliver, and it was actually recorded the week before this summit due to scheduling conflicts. Well, it was suggested to me as a guest by the kind sponsor of this episode and have several more episodes upcoming that I'm recording this week, Quantum Machines. Quantum Machines OPX 1,000 is tailored for large scale quantum computers offering unparalleled performance, scalability, and ready HPC integration, including an ultrafast interface to GPU and CPU accelerators for boosting quantum control.
Sebastian Hassinger:With hundreds of deployments worldwide, quantum machine solutions are trusted by quantum computer builders, research labs, and HPC centers. For more information, visit quantum-machines.co. And with that, let's get to my interview with professor Will Oliver. Welcome back to the podcast. We're joined today by Will Oliver, professor of physics from MIT, who's been very deeply involved in quantum computing for a very long time.
Sebastian Hassinger:I think one of the the OGs of the field in a lot of ways. MIT, of course, has been in I mean, Ike Chung, I think, built the first ion trap at MIT back in the early two thousands. Right? I mean, he sort of pivoted from NMR when he joined MIT. So the the history of the university goes way, way back.
Sebastian Hassinger:But today, we're gonna talk about some stuff that Will's been leading and involved with fluxonium qubits. So a new variety of superconducting qubits that have been turning out some really impressive results. So welcome, Will, and thanks for joining us.
Will Oliver:Yeah. Thanks, Sebastian. I'm really glad to be here and looking forward to the discussion.
Sebastian Hassinger:That's great. So so, you know, we're we're probably most familiar with the Transmon sort of design of superconducting qubits. That's what IBM and others have been building for quite some time. Yep. I think Fluxonium also came, if I'm not mistaken, from Yale.
Sebastian Hassinger:Think it was published a paper on on Flexonium sometime a few years back. But I think you guys you at MIT are the only ones who are sort of really putting a lot of effort into into, you know, improving the performance of those of those flexonian qubits. Right?
Will Oliver:Well, I wouldn't say that. I mean, you know, you're you're right that we certainly are putting a lot of effort in, but I I'd also, you know, recognize my many colleagues around the world who are also trying to do that. And of course, Vlad Manacharian is one of them and he was on the original paper with Michel, a grad student there. And he's continuing to look at it. There are a few other folks also doing it worldwide.
Will Oliver:But you're right. We're putting a lot of effort into it primarily because it has a lot of nice features which we can we can talk about.
Sebastian Hassinger:Yeah. Well, I mean, let's start with that. I mean, what differentiates the design of a flexonium qubit versus a a transmon?
Will Oliver:Yeah. It's quite different. Actually, you know, a fluxonium is a lot like the old flux qubit, persistent current flux qubit that Hans Mooij and Terry Orlando developed in the late nineties in the sense that it's it's got a double well potential. As you know, the Transmon, you know, its potential well looks a lot like a harmonic oscillator. It's only slightly anharmonic.
Will Oliver:But but the fluxonium looks a lot like a double well potential. It is. And so like all flux qubits, it's a loop, a loop of superconducting material, and it's interrupted by one what's called black sheep junction or small Josephson junction. The role of that junction is to let magnetic flux into or out of the loop. And then the rest of the loop is basically a large inductance.
Will Oliver:And that large inductance is realized by about a hundred or so Josephson junctions. Now they're bigger, those Josephson junctions, each one in area is bigger than the small black sheet. So we just consider them basically as linear inductors. Put a hundred of them in series, you have a pretty big inductance. And so that black sheep junction with a large inductor in a loop is what we call fluxonium.
Sebastian Hassinger:Okay. And so the it's still it's controlled by microwave pulses the way a trans bond is. Right? So those are those pulses are then creating the or or modifying the the the induced charge on the on the fluxonium qubit?
Will Oliver:Yeah. You can you can either drive it capacitively. I I didn't mention this, but there is a shunt capacitor often added to that small junction. Originally, wasn't, but but now we do that. So you can drive it that way called charge driving, but you can also flux drive it.
Will Oliver:You can have a microwave antenna coupled to the qubit and modulate the magnetic field in the loop, and that's another way to drive it.
Sebastian Hassinger:Okay. And and how are you doing at MIT?
Will Oliver:We do it both ways. We do it both ways. We do charge driving and flux driving. And in fact, we've done a comparison between those two. You know, I'm an old flux cubic guy, so I I like to flux drive everything.
Will Oliver:But most of the students and postdocs in the group these days, you know, they all grew up with the Transmon. And so everybody's a charge driver. And it's nice to have a bake off once in a while and see
Sebastian Hassinger:That's interesting. Yeah. Yeah. So the the the results you published in or was in the MIT technical review, I think, in January. I'm not sure when the original paper was published, but Yeah.
Sebastian Hassinger:It was covered in the in the magazine in January. The the one qubit gate fidelity was nearly five nines, which is kind of extraordinary for a superconducting qubit.
Will Oliver:Yeah. That was you know, we've been working for you know, along with the community for twenty years to make superconducting qubits better and better. And, of course, coherence is part of that story. But what really matters for implementing algorithms, you know, and the promise of quantum computing is to get high fidelity, right, that each operation works well. And so we actually had to go back to the drawing board and really dig into what's limiting the operational fidelity of these qubits, not just fluxonium, but any qubit.
Will Oliver:But we did it with fluxonium because it already started with a high coherence time. So, you know, most fidelities in superconducting qubits anyways are nearly coherence limited. Meaning if you make the coherence longer, the fidelity gets better. But we had already reached basically the coherence limit of fluxonium. I should mention it's, you know, some of the times that we see is T1 around one millisecond and T2 around three hundred to five hundred microseconds.
Will Oliver:And so starting there, the question was, you know, can we reach, the the coherence limit in fluxonium? And and so, that that led us to, you know, the techniques we developed in this paper to get it to five nines.
Sebastian Hassinger:Wow. That's amazing. And that was that using charge driving or flux driving?
Will Oliver:Well, we did it with both. And in in the end, the flux driving turned out to be a little bit better. And and for two reasons, which we could go into in more detail if you'd like. But but but but in a nutshell, we had coupled the charge line a little bit too strongly to the qubit. So we were driving it a little stronger than we would want to.
Will Oliver:And also a design aspect of the fluxonium or feature of fluxonium you could say is that the charge matrix elements are pretty strong all the way up the spectrum. And so you want to drive zero one and you can do that pretty well because of the high anharmonicity of fluxonium, but you still have a non negligible leakage to higher energy levels. And and that leakage at the five nines level is, you know, you know, you can't ignore it.
Sebastian Hassinger:Yeah. Right. Right. Right. Right.
Sebastian Hassinger:Interesting. And and you said you also that was a a one cubic gate, but you've you've got quite high fidelities on two cubic gates as well subsequently. Right?
Will Oliver:Yeah. Well, actually, we started with the two qubit gate. So this device has two qubits, two fluxonium qubits, and we used a Transmon as a coupler between them. Mhmm. So we could basically turn on and off the interaction.
Will Oliver:And in that gate, and this was by, Leon Ding, who who graduated in 2023 with his PhD. So he led that effort and, you know, he was able to get 99.92 to three, something like that on this gate over several hours. So so that was the device. And then the follow on work was, okay, well, let's look at the single qubits and see how good we could do. So then Leon and also David Rohwer, who led this effort, recently graduated as well, looked at the single fluxoniums and then, you know, did this experiment to get almost five nines.
Sebastian Hassinger:Wow. That's cool. And and so you're you're talking about a test device, an experimental qubit device with just a couple of qubits on it. Is there like, how do you think the design of fluxonium will lend itself to larger scale QPUs?
Will Oliver:Yeah. So the one the one thing about fluxonium is that it's it's somewhat sensitive to capacitance. And in a sense, there's a capacitance budget that one has to respect. Now because of that, I think at a cursory level, people decided, fluxonium, you know, it's interesting at the one or two qubit level, but it'll never scale because of this capacitance limitation that you have. We worked through it, came up with some designs such that we we can actually couple them in a north, south, east, west manner.
Will Oliver:So you could make a surface code out of this. I think that, you know, there is a regime of parameter space in which Fluxodium will scale, and that turned out to be the genesis of a startup company that came out of our group called Atlantic Quantum. Yeah. So we certainly did our homework before launching that.
Sebastian Hassinger:Yeah. I I assume so. Yeah. One qubit's not gonna get you very far. No.
Sebastian Hassinger:No. No. That's right. Recent press releases notwithstanding. But
Will Oliver:Zero qubit. Yeah.
Sebastian Hassinger:Yeah. So yeah. So Atlantic Quantum is is the the name of the startup. I think it's still sort of in in development mode. Right?
Sebastian Hassinger:I haven't seen any, any launch plans or anything of of a, I think, device. But you must be sort of heading in that direction. You've got sort of a plan to get something, into the the hands of potential customers.
Will Oliver:Yeah. That's right. So we did our seed round almost three years ago, I think early twenty twenty two. '13 million raise, and then we got about that much again in, nondilutive government funding. So this year we're starting an A raise, but right now the main product is IP and how one can build a scalable fluxonium based quantum computer with a focus on driving down the cost per qubit.
Will Oliver:That that's the main feature. Turns out fluxonium has a rather low frequency compared to most qubits. You can operate it around a hundred megahertz, 200 megahertz, whereas, you know, typical transmons might be around five gigahertz. That low frequency turns out to have some very, very nice features and and that's where our IP is really centered in terms of how you can control it in somewhat nonstandard ways, but ways in which, you know, you can co locate cryogenic electronics and thereby reduce the cost per qubit substantially. So anyways, that that's the direction that Atlantic Quantum's going.
Sebastian Hassinger:That's really cool. So you're saying essentially a lot of the I mean, with TransMons, you've got to operate, as you said, sort of in the five gigahertz range. Yeah. That's great. And that means you've got to put a a microwave lead down in the dill fridge for every cubit at this point.
Sebastian Hassinger:They we haven't figured out how to multiplex as far as I know. Good point. Yeah. And so that's a huge space space constraint on on what you can fit in a dill fridge. And also, as you said, it's very expensive on a per cubic basis to for the control architecture.
Sebastian Hassinger:So so you think fluxonium would actually be able to have that the con some of the controls actually in the fridge with the with the cubits?
Will Oliver:Yep. We do. And and that's really what, Atlantic's focused on right now. And, you know, you can drive down the cost a lot because it as you just said, right, a five gigahertz generator is pretty expensive, burns a lot of power. It's basically a little computer at room temperature.
Will Oliver:Right? It's got an IC inside. Once you reduce the frequency dramatically, then that opens up a lot of new doors that you can, you know, start thinking about putting a lot of this stuff down at cryogenic temperatures.
Sebastian Hassinger:Interesting. That's really cool. That potentially would mean you'd be able to fit more cubits in a single dill fridge as well because you wouldn't have to have one of those big leads all the way from room temp down to to the millikelvin range.
Will Oliver:Yep. That's exactly great. Yep. That's exactly right.
Sebastian Hassinger:So are you are you building your own sort of control stack as well then because of the the sort of the unique characteristics that you're designing around?
Will Oliver:We do. We do have teams of people now that are focused on what that control looks like, both in terms of design and emulation of the signals, testing those signals, designing the cryogenic electronics as well.
Sebastian Hassinger:That's really cool. That's really cool. So so then what I mean, can you sort of project the the sort of whatever the the the target for how many cubits you might be able to fit in in a single Dell fridge with this type of setup?
Will Oliver:Yeah. I I you know, it's hard to say because, you know, there's so many different types of dill fridges. And, really, if you needed more power, you just would make it you know, add another mixing chamber and make it twice as big and you've got twice as much cooling power. So I'm hesitant to say, you know, a concrete number, but but I can I can say with certainty that it's a lot more than what we're currently doing with all those coaxes coming down?
Sebastian Hassinger:Right. Right. Yeah. Yeah. That stands to reason.
Will Oliver:There's big win per qubit. And and the more you can do it, you think about it. Right? Today, we're probably close to 5 or even $10,000 per qubit. And we know we're being able do qubits.
Will Oliver:Yeah. How many of those computers can we really build?
Sebastian Hassinger:I know. I know. The the math starts to get pretty daunting when you start multiplying by, you know, like surface code ratios and and, you know, the number of logical cubits you need for something like shores. It starts sounding like a very, very expensive computer. Indeed.
Sebastian Hassinger:So that's that's really cool. So are you you mentioned surface code. Is that sort of what what the the play is then is to build a chip at scale that can implement surface code for error correction and produce sort of logical qubits out of out of those. I mean, obviously, because the fidelity is so high, your ratio is actually gonna be quite attractive, right, of physical qubits to logical qubits?
Will Oliver:Yeah. That's right. So so we're part Atlantic Quantum as well as MIT campus is part of the IARPA Entangled Logical Cubits Program or ELK as it's called. And so our goal there is to do one and two, you know, ultimately two cubic gate operations. And we'll do that with the surface code.
Will Oliver:Fluxonium is in a surface code architecture. And this is really you know, it's a great platform because we know we're gonna need error correction. Right? I think, you know, the community's been trying for a long time to come up with something in the NISC era that's useful, and and we we still may find it. I'm not gonna say it's not gonna happen.
Will Oliver:But if you take a step back and say, okay, what's really gonna take us to commercial value and realizing that commercial promise, it almost certainly is gonna be an error corrected machine just because of the depth of the algorithm that we require. And so we're focused on, you know, improving the fidelity of each qubit and then scaling up. So make better qubits and then scale them up and, you know, starting with surface codes and error correction is a great idea. I do have, you know, some students at MIT who are thinking about QLDPC codes, quantum low density parity check codes and long range couplers and things like that. And, you know, when those types of elements become, you know, become real, then, you know, companies can adopt them and and try other codes.
Will Oliver:But right now, the surface code matches the nearest neighbor connectivity that we get in a superconducting processor quite well. Yeah.
Sebastian Hassinger:Yeah. Yeah. Yeah. And, I mean, Google's result from the end of last year, you know, of sort of the below threshold was really I mean, it was such an incredible step. Unfortunately, often misconstrued in in the popular press.
Sebastian Hassinger:Yeah. That's kinda I mean, that's one of the motivations for this podcast is to try to to, to add a little bit more nuance to this to the, the news. But, I mean, you know, effectively, they proved that the surface code is, an efficient way to like, the more physical cubits you add, the the longer you can actually operate in, you know, fault tolerant mode. Yeah. And it's, you know, it's it was it was definitely good validation for people like yourself who are are planning on implementing surface code.
Sebastian Hassinger:Yeah. Is there when when you think about the the the way you're gonna lay out the chip, like you said, next nearest neighbor, the the sort of the laid out in a cross. Right? Like a lattice? Yeah.
Sebastian Hassinger:That's right.
Will Oliver:That's right.
Sebastian Hassinger:North, south, east, west. Is do you is there any major difference in the way that you're gonna have to implement service code compared to the the the Google approach? Because I think that was the lattice as well. Right?
Will Oliver:That's right. Yeah. That that by the way, the Google result was really fantastic and and so glad to see that. I mean, it was the first time on any platform that that this had been experimentally shown. And, you know, this is the side of the line we gotta be on.
Will Oliver:So good to see. I think, you know, there there, of course, will be differences in the details, but I think this general pattern of a lattice of qubits and coupling north, south, east, and west that this is this is what it will look like. Yeah. That's
Sebastian Hassinger:right. Right.
Will Oliver:Yeah.
Sebastian Hassinger:That's really cool. And so do you you know, you mentioned other approaches that are are being experimented with by other groups within MIT. It's you know, I I it feels like this we're still in this stage where it's like we should be exploring all the possibilities because no one can sort of claim to have a a clear path. Is there are there other potential modalities that you're also interested in?
Will Oliver:Absolutely. So even in our own group, we've got currently 12 DIL fridges, but two of them are for semiconductor qubits. So working with Intel and HRL And, you know, what we can bring to semiconductor qubits is perhaps, you know, what we've learned from semi superconducting, like superconducting couplers or something like that. A lot of three d integration work that we've done, you know, and trying to understand, you know, what parts of that can be brought to bear for semiconductors to help out that modality. But semiconductor qubits are really starting to stand up in the last few years and lots of fantastic progress, you know, for all different types.
Will Oliver:There's so many different types even.
Sebastian Hassinger:Yeah. Yeah. That's a it's true. It's sort of opening an entirely new can of worms in terms of the the diversity. I mean, so is it you mentioned Intel.
Sebastian Hassinger:So this is this is in was it? Tunnel Tunnel Top? Is that what they called their chip, I think? Something that they had a a 12 qubit experiment.
Will Oliver:Yeah. That's the one. So 12 qubits in
Sebastian Hassinger:qubit chip.
Will Oliver:Yeah. And what's nice about that, I really like that design because you could operate each one as 12 single qubits or you could do six double dots, or you could do four triple dots. Right? And so, you know, you could really test all of these different modalities against one another with the same, you know, fundamental qubit design, which I find to be pretty interesting. And, so that's one of them and this is, you know, enabled through the LPS foundry program.
Will Oliver:Also with HRL, also part of the LPS foundry program, looking at their version of triple dots specifically. So so we're just starting. We don't have any, you know, major results yet, but we are dipping our toes in semiconductor qubits because I think, you know, like like we just said, they're starting to stand up and it's pretty interesting.
Sebastian Hassinger:Well and certainly, I mean, intuitively, it feels like if you can crack the code on doing quantum dots or spin qubits in silicon, then we've got you certainly have the know how for doing silicon at scale. Like Yeah. Well, all of a sudden, it becomes, like, the scaling question sort of becomes, easier, I think. I mean, at least that's what I would imagine.
Will Oliver:Well and, you you know, you I think you hit the nail on the head in the sense that all of these platforms have superpowers or strengths, and they also have weaknesses. Right? And so clearly, you know, semiconductor qubits have that superpower, which is that you if you can make them in the foundries, already exist, you've already got a leg up on scalability, you know. And superconducting qubits, I would say, also fall in that camp. You know, and even photonic qubits.
Will Oliver:Right? A lot of the integrated photonics can be made in these. So so it's not just the domain of semiconductor qubits, but, of course, on the other hand, they are indeed the closest to that. And the three d integration that we're going to require to in any of these platforms, of course, has been, you know, developed quite extensively for for semiconductor chips and, you know, chiplets. And we're we're seeing it more and more as we've gotten beyond Moore's law.
Will Oliver:It doesn't mean it's the end of the road map. It just means, okay, instead of making the chips faster, we're just gonna three d integrate them more and more. Right.
Sebastian Hassinger:Right. Right. That's really interesting. And I I think MIT is part of the the c two q a. Right?
Sebastian Hassinger:The the DOE NQI center?
Will Oliver:Yeah. We're part of the Brookhaven one. Right. And we're also part of the Berkeley Sandia one called QSA. Cool.
Will Oliver:So CTQA and QSA, we're members of both of those.
Sebastian Hassinger:Right. I I was just curious because CTQA sort of has the the co design kind of ethos sort of embedded in its in its mission, meaning, like, sort of algorithmic design or experimentation sort of interacting more with experimental approaches to qubits and architectures. It was has there been any of that sort of interplay between sort of the theorists and the experimentalists in the in the the work you've done with the flexionium?
Will Oliver:Yes. There has been. We've we've talked with folks at C2QA and theorists there about experiments that we could do with fluxonium, also with TransMons. And that turns out, you know, that's a big part of that program as well. And then, you know, to be fair to QSA as well, you know, co design is a big part of that one and, you know, also the theorists in the QSA team.
Will Oliver:So but but there it's less focused on for both of these centers, it's less focused on I'm gonna use fluxonium and not Transmon or Transmon and not fluxonium. It's more about, you know, what can we achieve and what's the best cue to achieve a particular goal, and that's, you know, derivative of this co design mentality.
Sebastian Hassinger:Right. Right.
Will Oliver:Yeah.
Sebastian Hassinger:That's really interesting. Yeah. It does it does feel like there may be some kind of interim stage where we have multiple modalities in in single system architectures to to that leverage, as you said, the the superpowers and try to, you know, make up for the the the the weaknesses of each of these modalities when you look at them one at a time. Do you do you I mean, is there that kind of of integrated architecture thinking happening at all m I at MIT with all the different modalities you've got in play?
Will Oliver:Well, I would say that, you know, within say the cryogenic ones like superconductors and semiconductors, that is an area we're exploring. We we don't know yet if it's, you know, going to pay off in the end because, you know, there's no free lunch. Gain some of the superpower, but we also gain some of, you know, some of the part that's not so good. And so which one wins in the end? We'll have to see.
Will Oliver:Of course, MIT is also doing trapped ions, neutral atoms, some photonics work. We haven't really tried to say combine yet superconductors and say the natural atoms, mostly because the time scales are so different. And and usually, you're limited by the slower time scale, which means, you know, by the time the superconducting qubit can give its information over to a trapped ion, it's already lost the information because superconductors are fast and they lose their information fast. So Yeah.
Sebastian Hassinger:Yeah. I guess, I mean, I just wonder from a certain perspective that slowness and long coherence times on on atoms almost looks like, you know, storage, like, to a superconducting qubit.
Will Oliver:It does. And, you know, people have suggested this and, you know, I'm I'm definitely open to that idea. The the roadblock that I keep hitting, at least mentally as I do a, you know, mental experiment is that, you know, you've got a fast processor of superconducting qubits and then you wanna put something into memory in a trapped ion. And the ions are about a thousand times slower.
Sebastian Hassinger:Right.
Will Oliver:And so as you as the superconductors try to give that, you know, quantum information over to the trapped ion, they lose it before the trapped ion can get it. So then you say, well, let's make faster trapped ions. That'll work. But then if you can make them fast as the superconductors, just use the trapped ions. They're really good.
Will Oliver:So
Sebastian Hassinger:Good point. Good point. I didn't think of that. Of course, it's the the data intro you know, input and output would be the the gating factor.
Will Oliver:Yeah. So but but, again, I think it's good to think through these things and because, you know, engineers are clever. I I will almost never say that something won't work.
Sebastian Hassinger:Right.
Will Oliver:I can only say what I'm currently thinking and why I have this mental block on it right now, but it's possible. Right? Let's Yeah. Let's keep chewing on it.
Sebastian Hassinger:That's what I find so fascinating about this whole space is that that this hardware is being developed, like, you know, in this sort of knife's edge balance between applied science, like fundamental research, and really, really cutting edge engineering. It's, like, incredible, sort of, like, the peak of both of those kind of, disciplines and skill sets working in conjunction is something to see.
Will Oliver:Yeah. Yeah. No. You're absolutely right. And, you know, probably for the first ten or twenty years of this development, it was a lot of quantum science, and that needs to continue.
Will Oliver:But the quantum engineering, as we call it now, that needs to stand up. And I think over the last few years, a lot more attention has been given to it. But, you know, we still we still need to, as an engineering discipline, develop the abstractions that allow us to then go forward and engineer quantum systems. I think writing the textbooks, developing the classes, you know, the courses, you know, that that people can take, the professional development courses, these are things that we're focused on at MIT because, you know, a, that's our job. We're supposed to do that.
Will Oliver:But but b, it's gonna be so important for the future. And, you know, the idea that we're gonna build a large scale quantum computer at a university is kind of, you know, it's let me say, it's probably not gonna happen. That's the job of a university. But what sorry, that's the job of industry. Yeah.
Will Oliver:But but what a university can do is, you know, the fundamental basic engineering and the principles and learning how to scale. And then industry can take those that learning and then apply it to actually scale.
Sebastian Hassinger:Yeah. Excellent. Yeah. And that's I mean, hopefully, Atlantic Quantum can can be an example of exactly that kind of of handoff of the the fundamentals, the the roots of the the the learnings of the from the university lab into creating a commercial product.
Will Oliver:Yeah. That's certainly the idea.
Sebastian Hassinger:Yeah. That's great. Well, thank you so much for joining us, Will. This has been really, really interesting. I look forward to reading more about Flexonium and Atlantic Quantum and, of course, all of the incredible work that's going on at MIT.
Sebastian Hassinger:So thank you.
Will Oliver:Thank you, Sebastian. Really enjoyed it. Take care.
Sebastian Hassinger:Thank you to professor Will Oliver. Thanks to Quantum Machines for their support. Stay tuned for more from the twenty twenty five Global Summit, and thanks to APS for their generosity. If you have questions or comments, follow the show on blue sky at new quantum era and reply to this episode's post. To find prior episodes, show notes, transcripts, or to preview the book I'm writing on the topic, go to newquantumera.com.
Sebastian Hassinger:Thank you for listening. And if you wanna support the show, please tell your friends. And if you have suggestions for topics or guests, please let me know on Blue Sky. Thanks again.
Creators and Guests
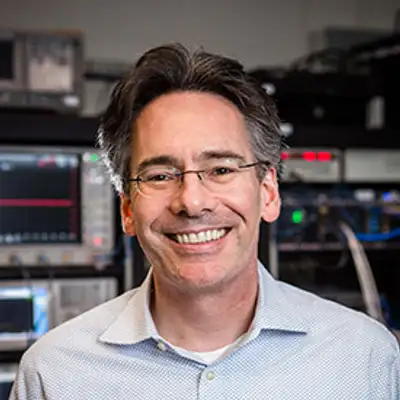